ISSN: 0973-7510
E-ISSN: 2581-690X
https://dx.doi.org/10.22207/JPAM.13.1.72 | © The Author(s). 2019
Nosocomial or hospital acquired infections are acquired by the patients during hospital stay. Bacteria were found to be responsible for the majority of infections followed by fungi, viruses, and protozoa. Literature has demonstrated that hospitals with infection control guidelines help reduce infection rates. We therefore isolated and compared common nosocomial organisms causing infections previous to and after the implementation of infection control measures to control healthcare-associated infections in our hospital setting. Strategy for infection control in our hospital setting was devised in the year 2010. These guidelines were frequently revised to improve the compliance of infection control measures. Infection control nurse helped record all the positive cultures in a surveillance format. This data on the number of isolates recovered from the cultures was documented and compared each year to check for the compliance of the infection control guidelines to reduce microbial isolates causing infections. Of the recovered 866 isolates from microbial cultures known to be causing nosocomial infections, 77.7% were gram-negative bacteria, 13.2% were gram-positive bacteria and 9% were fungi. A decrease in the number of microbes causing infections was observed after the implementation of infection control measures, with only 3.4% of the gram-positive microbes, 9.6% of the gram-negative microbes and 3.8% of the fungi being identified in the year 2017. Our study demonstrated that effectively devised infection control measures help reduce the number of organisms causing nosocomial infections further resulting in decreased infection rate.
Nosocomial Infections; Health Issues; Infection Control; Bacteria; Hospital Stay.
The term “mycorrhiza” was coined by A. B. Frank1 which literally means ‘fungus-root’ and describes the mutualistic association existing between a group of soil fungi and higher plants. Arbuscular Mycorrhizal Fungi (AMF) form mutualistic symbioses with roots of over 80% of known plant species2 including bryophyte, pteridophyte, gymnosperm and angiosperm. AMF enhance the uptake and transfer of nutrients with low mobility to plants such as, phosphorus (P), Zinc (Zn), Nitrogen (N) and Iron (Fe)3, 4. In addition to absorption of mineral nutrients, AMF stabilize soil structure and enable plants to survive under stress conditions such as drought, saline and toxic soil5-7. AMF also influence soil processes, plant biodiversity and sustainability of terrestrial ecosystems8, 9.
The coalmine overburden (OB) spoils vary widely in their physical, chemical and biological characteristics than natural soil, which affect plant establishment, survival and growth. AMF play an important role in the establishment of vegetation and initiation of nutrient cycling for the development of self-sustaining ecosystem in overburden spoils and other mined out areas10, 11. Fresh OB spoils are usually dry, polluted with heavy metals and lack viable mycorrhizal population which affect the development of vegetation. Under these conditions, AMF can enhance the mineral absorption and draught tolerance of plants for their growth and survival. A thorough and systematic study on the occurrence of AMF species, spore density, species diversity and composition in abandoned coal mine overburden spoils will, therefore, be of great help in implementing successful reclamation programmes.
One of the most widely used methods for AMF identification is through morphological characteristics of spores collected either directly from the field12 or from trap cultures13. Field collected spores, in some circumstances, lack informative taxonomic characteristics impairing a more accurate identification. Moreover, in the field conditions, AMF spores may be less in number or some species may be absent at the time of sampling even though they may be present within roots14. Alternatively, establishment of trap cultures produce clear and healthy spores which greatly improves the assessment of species composition in an ecosystem15. However, all AMF species present in the field cannot be assessed through trap culture since, on one hand sporulation of some species is affected by host plant chosen for trapping13 whereas on the other hand it promotes the sporulation of cryptic AMF species which were not sporulating at the sampling time or field conditions16. Despite of that, trap cultures have been widely used to assess AMF diversity and isolate indigenous fungi17.
Since AMF can be utilized for successful establishment of plants in highly disturbed habitats, more information on their ecology is required. It is essential to know the AMF species present in a particular soil environment before they can be studied for reclamation process. For the present study soil samples and root fragments from abandoned coal mine overburden spoils of different age groups (2 years, 10 years and 15 years abandoned sites and an un-mined site) were collected from East Jaintia Hills District, Meghalaya, India.
Propagation of AMF spores through trap culture
The methods of AMF trap culture were followed from INVAM (http://invam.caf.wvu.edu). Field soil and root fragments from each study site that served as inocula for AMF trap cultures were collected in separate sterilized plastic bags and transported to the laboratory. Roots were cut into small fragments and mixed thoroughly with the associated soil. This chopped blend was mixed with autoclaved coarse sand (1:1 v/v). Oryza sativa L. (upland variety) and Zea mays L. were used as trap plants. These species were chosen as they are well-known AMF host plants frequently used for trap cultures. Seeds were surface sterilized with sodium hypochlorite (10% v/v) for 10 min and thoroughly rinsed with sterilized water. Approximately 15-20 seeds of Oryza sativa L. and 6-7 seeds of Zea mays L. were evenly sown on 25cm diameter plastic pots containing 1.5kg of inoculum and autoclaved coarse sand (1:1 v/v) and monitored in greenhouse condition. They were watered whenever required.
AMF colonization assessment
After 4 months, all trap plant species were removed from the plastic pots and roots were separated for evaluation of AMF colonization. For each species, root samples were washed thoroughly in tap water, then processed and stained with 0.05% Trypan blue18. Root tissues were quantified for colonization by magnified intersections method19 and converted to percentage.
AMF spore analysis
AMF spores were extracted from 100g soil sample by wet sieving and decanting method20. AMF spores were picked up with needle in polyvinyl alcohol-lactoglycerol with Meltzer’s reagent on a glass slide. The complete and broken spores were examined using a compound microscope, Olympus. Spore identification was done based on morphological descriptions published by INVAM (http://invam.caf.wvu.edu) and AMF phylogeny (www.amf-phylogeny.com).
Data analysis
Means and standard errors were calculated. Spore density and species richness were expressed as number of AMF spores and number of AMF species in 100g soil samples. Relative abundance, isolation frequency, Shannon-Wiener diversity index, Simpson’s dominance index and Pielou’s Evenness index were calculated.
Zea mays L. showed variation in AMF colonization in the roots whereas colonization in the roots of Oryza sativa L. did not show much variation. In Z. mays AMF colonization ranged from 60.15% (2 years abandoned site inoculum) to 93.09% (un-mined site inoculum). In O. sativa AMF colonization ranged from 78.71% (2 years abandoned site inoculum) to 82.07% (15 years abandoned site inoculum) (Fig. 1). In both the trap plants AMF spore density is least in 2 years abandoned site derived inoculum and highest in un-mined site derived inoculum (82 spores/100 g soil and 155 spores/ 100g soil respectively in Z. mays and 115 spores/100g soil and 204 spores/ 100g soil respectively in O. sativa) (Fig. 2) .
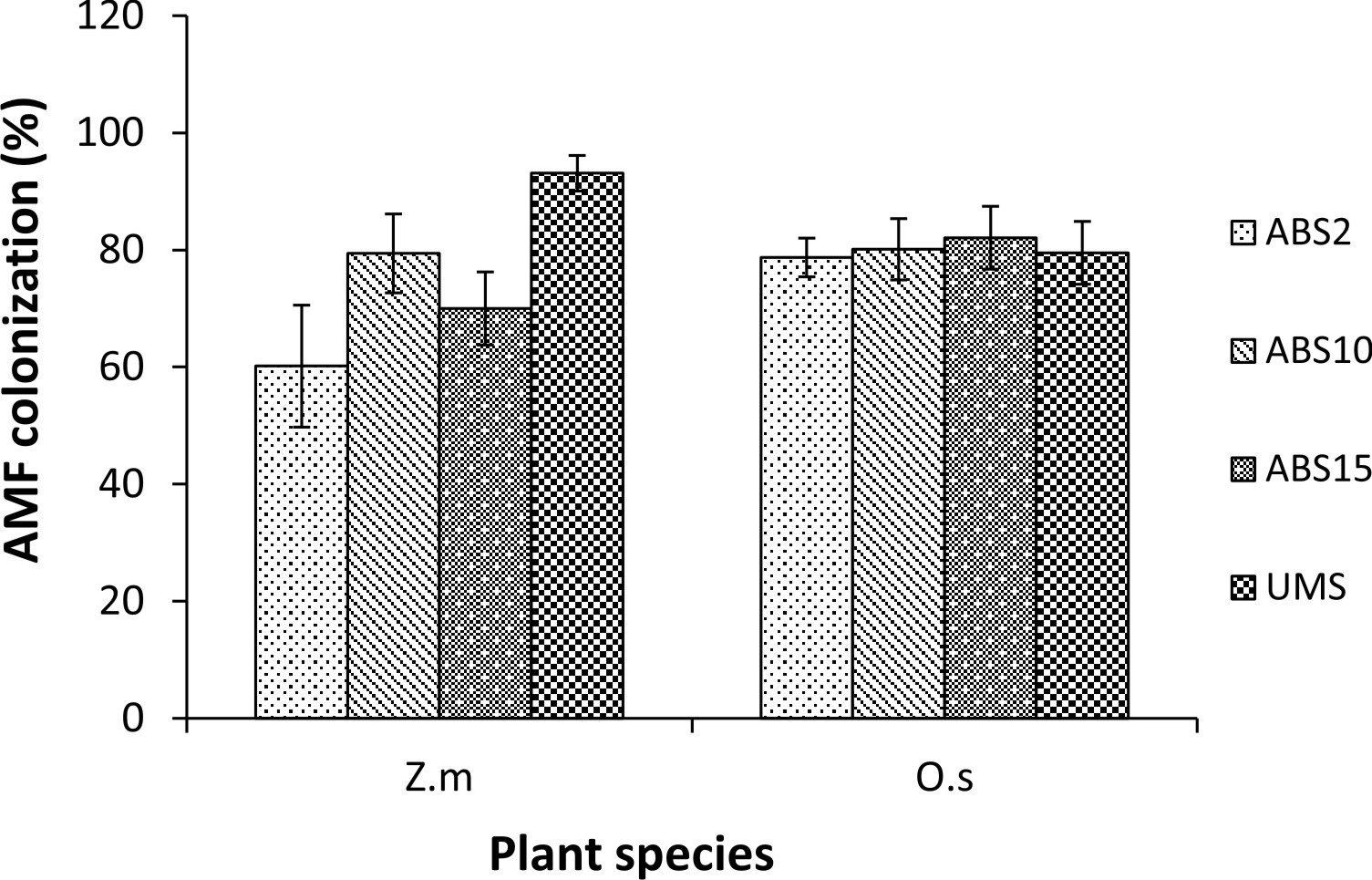
Fig. 1. AMF colonization (%) in Zea mays L. (Z.m) and Oryza sativa L. (O.s) used as trap plants set up with study sites derived inocula.
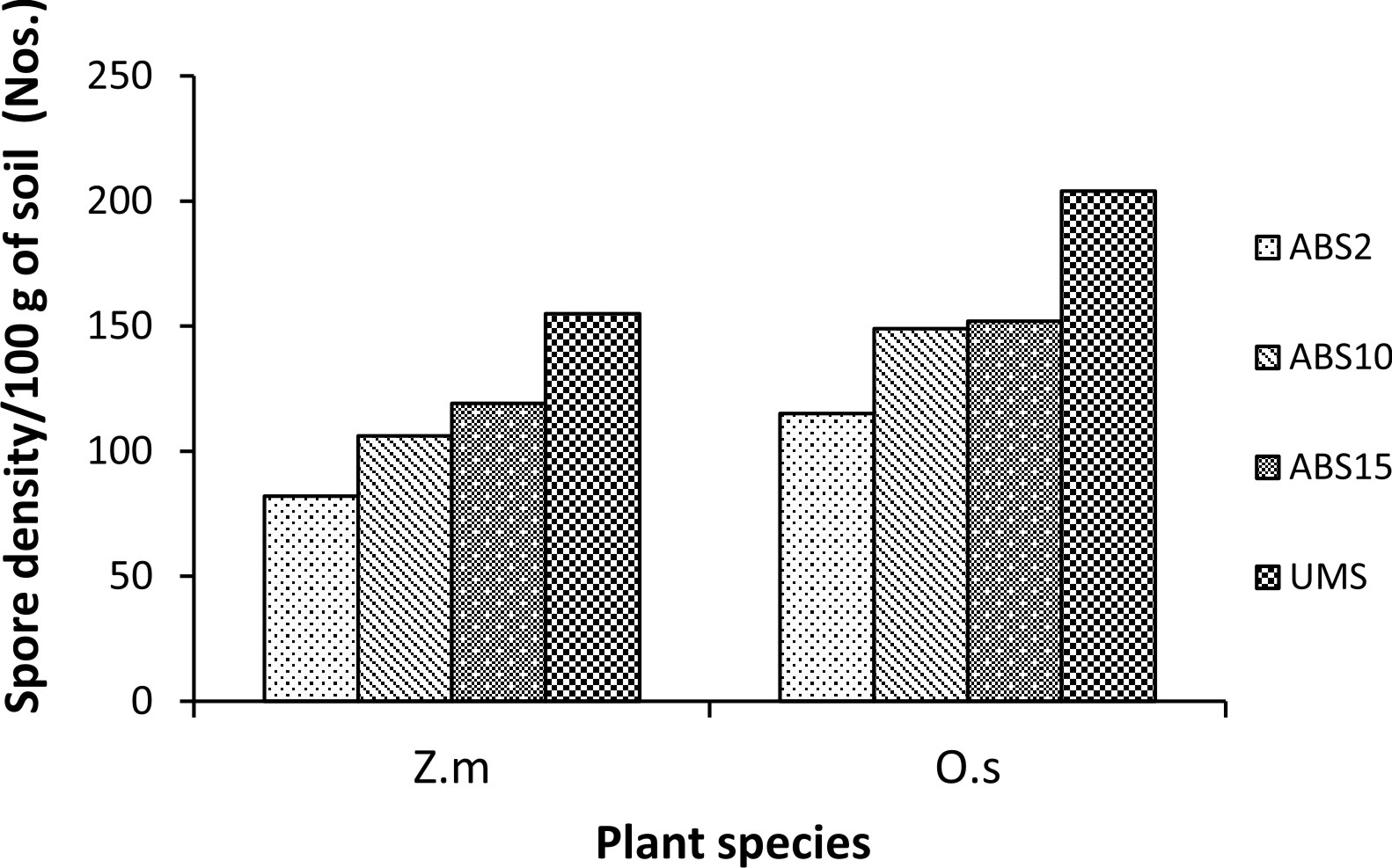
Fig. 2. Percentage of gram-positive, gram-negative and fungal organisms isolates recovered from the cultureAMF spore density in trap culture set up with study sites derived inocula where Zea mays L. (Z.m) and Oryza sativa L. (O.s) are used as trap plants.
With 2 years abandoned site derived inoculum, 10 AMF species were isolated from Z. mays trap culture and 13 AMF species from O. sativa trap culture. Funneliformis geosporus has the highest relative abundance in Z. mays (18.29%) and Acaulospora morrowae has the highest relative abundance in O. sativa (20.87%) (Table 1). With 10 years abandoned site derived inoculum, 12 AMF species were isolated from Z. mays trap culture where Acaulospora mellea and Acaulospora morrowiae has the highest relative abundance (11.32%) and 16 AMF species were isolated from O. sativa trap culture where F. geosporus (18.12%) has the highest relative abundance (Table 2). With 15 years abandoned site derived inoculum 14 AMF species were isolated from Z. mays trap culture and 18 AMF species from O. sativa trap culture and with un-mined site derived inoculum, 17 AMF species were isolated from Z. mays trap culture and 22 AMF species from O. sativa trap culture. F. geosporus has the highest relative abundance in both the trap plants set up from15 years abandoned site inoculum and un-mined site inoculum (Table 3 and 4).
Table (1):
AMF species isolated from trap cultures (2 years abandoned site derived inoculum) with their relative abundance and isolation frequency (IF) using Z. mays and O. sativa as trap plants
Sl. No. | AMF species | Relative abundance (%) | IF % | |
---|---|---|---|---|
Z. mays | O. sativa | |||
1 | Acaulospora capsicula Blaszk. | 10.98 | 6.09 | 100 |
2 | Acaulospora delicata Walker, Pfeiffer & Bloss | 10.98 | 2.61 | 100 |
3 | Acaulospora foveata Trappe & Janos | – | 2.61 | 50 |
4 | Acaulospora laevis Gerd. & Trappe | 9.76 | 6.09 | 100 |
5 | Acaulospora mellea Spain and Schenck | 13.41 | 13.04 | 100 |
6 | Acaulospora morrowiae Spain and Schenck | 10.98 | 20.87 | 100 |
7 | Claroideoglomus etunicatum Walker & Schuessler | – | 2.61 | 50 |
8 | Claroideoglomus luteum Walker & Schuessler | 8.54 | 10.43 | 100 |
9 | Funneliformis geosporus Walker & Schuessler | 18.29 | 17.39 | 100 |
10 | Funneliformis verruculosum Walker & Schuessler | 12.20 | 1.74 | 100 |
11 | Glomus multicaule Gerd. & Bakshi | 1.22 | 1.74 | 100 |
12 | Rhizophagus clarus Walker & Schuessler | – | 12.17 | 50 |
13 | Rhizophagus intraradices Walker & Schuessler | 3.66 | 2.61 | 100 |
Table (2):
AMF species isolated from trap cultures (10 years abandoned site derived inoculum) with their relative abundance and isolation frequency (IF) using Z. mays and O. sativa as trap plants.
Sl. No. | AMF species | Relative abundance (%) | IF % | |
---|---|---|---|---|
Z. mays | O. sativa | |||
1 | Acaulospora capsicula Blaszk. | 8.49 | 5.37 | 100 |
2 | Acaulospora delicata Walker, Pfeiffer & Bloss | – | 6.04 | 50 |
3 | Acaulospora foveata Trappe & Janos | – | 4.03 | 50 |
4 | Acaulospora lacunosa Morton | 5.66 | – | 50 |
5 | Acaulospora mellea Spain and Schenck | 11.32 | 10.07 | 100 |
6 | Acaulospora morrowiae Spain and Schenck | 11.32 | 10.07 | 100 |
7 | Claroideoglomus etunicatum Walker & Schuessler | 6.60 | 5.37 | 100 |
8 | Claroideoglomus luteum Walker & Schuessler | 8.49 | 9.40 | 100 |
9 | Funneliformis geosporus Walker & Schuessler | 24.53 | 18.12 | 100 |
10 | Funneliformis verruculosum Walker & Schuessler | 2.83 | 6.04 | 100 |
11 | Glomus glomerulatum Sieverding | – | 2.68 | 50 |
12 | Glomus macrocarpum Tul. & Tul. | – | 4.03 | 50 |
13 | Glomus multicaule Gerd. & Bakshi | 2.83 | 2.01 | 100 |
14 | Glomus sp.1 | – | 4.03 | 50 |
15 | Rhizophagus clarus Walker & Schuessler | 8.49 | 8.05 | 100 |
16 | Rhizophagus intraradices Walker & Schuessler | 6.60 | 2.01 | 100 |
17 | unidentified sp.1 | 2.83 | – | 50 |
18 | unidentified sp.2 | – | 2.68 | 50 |
Table (3):
AMF species isolated from trap cultures (15 years abandoned site derived inoculum) with their relative abundance and isolation frequency (IF) using Z. mays and O. sativa as trap plants.
Sl. No. | AMF species | Relative abundance (%) | IF % | |
---|---|---|---|---|
Z. mays | O. sativa | |||
1 | Acaulospora capsicula Blaszk. | – | 5.92 | 50 |
2 | Acaulospora delicata Walker, Pfeiffer & Bloss | 6.72 | 4.61 | 100 |
3 | Acaulospora foveata Trappe & Janos | – | 4.61 | 50 |
4 | Acaulospora lacunosa Morton | 3.36 | 3.29 | 100 |
5 | Acaulospora laevis Gerd. & Trappe | 3.36 | 4.61 | 100 |
6 | Acaulospora mellea Spain and Schenck | 7.56 | 9.21 | 100 |
7 | Acaulospora morrowiae Spain and Schenck | 11.76 | 8.55 | 100 |
8 | Acaulospora rehmii Sieverding & Toro | – | 2.63 | 50 |
9 | Claroideoglomus etunicatum Walker & Schuessler | 5.88 | 4.61 | 100 |
10 | Claroideoglomus luteum Walker & Schuessler | 9.24 | 9.87 | 100 |
11 | Funneliformis geosporus Walker & Schuessler | 24.37 | 17.11 | 100 |
12 | Funneliformis verruculosum Walker & Schuessler | 4.20 | 6.58 | 100 |
13 | Glomus ambisporum Smith & Schenck | – | 1.32 | 50 |
14 | Glomus macrocarpum Tul. & Tul. | 5.04 | 2.63 | 100 |
15 | Glomus multicaule Gerd. & Bakshi | 2.52 | 1.97 | 100 |
16 | Glomus rubiforme Almeida & Schenck | 3.36 | – | 50 |
17 | Rhizophagus clarus Walker & Schuessler | 6.72 | 6.58 | 100 |
18 | Rhizophagus intraradices Walker & Schuessler | 5.88 | 3.95 | 100 |
19 | Unidentified sp.1 | – | 1.97 | 50 |
Table (4):
AMF species isolated from trap cultures (un-mined site derived inoculum) with their relative abundance and isolation frequency (IF) using Z. mays and O. sativa as trap plants.
Sl. No. | AMF species | Relative abundance (%) | IF % | |
---|---|---|---|---|
Z. mays | O. sativa | |||
1 | Acaulospora capsicula Blaszk. | 6.45 | 6.37 | 100 |
2 | Acaulospora delicata Walker, Pfeiffer & Bloss | 4.52 | 4.90 | 100 |
3 | Acaulospora foveata Trappe & Janos | 3.87 | 5.39 | 100 |
4 | Acaulospora lacunosa Morton | 5.81 | 2.94 | 100 |
5 | Acaulospora laevis Gerd. & Trappe | 3.87 | 2.94 | 100 |
6 | Acaulospora mellea Spain and Schenck | 7.74 | 5.88 | 100 |
7 | Acaulospora morrowiae Spain and Schenck | 6.45 | 6.86 | 100 |
8 | Acaulospora rehmii Sieverding & Toro | – | 2.94 | 50 |
9 | Claroideoglomus etunicatum Walker & Schuessler | 6.45 | 4.41 | 100 |
10 | Claroideoglomus luteum Walker & Schuessler | 8.39 | 8.82 | 100 |
11 | Funneliformis caledonium Walker & Schuessler | – | 1.96 | 50 |
12 | Funneliformis geosporus Walker & Schuessler | 14.19 | 11.76 | 100 |
13 | Funneliformis verruculosum Walker & Schuessler | 3.87 | 5.88 | 100 |
14 | Glomus ambisporum Smith & Schenck | – | 1.96 | 50 |
15 | Glomus glomerulatum Sieverding | 3.23 | 2.45 | 100 |
16 | Glomus macrocarpum Tul. & Tul. | 6.45 | 2.94 | 100 |
17 | Glomus microcarpum Tul. & Tul. | – | 1.47 | 50 |
18 | Glomus mosseae Gerd. & Trappe | 3.23 | – | 50 |
19 | Glomus multicaule Gerd. & Bakshi | 2.58 | 1.96 | 100 |
20 | Glomus rubiforme Almeida & Schenck | 4.52 | 3.92 | 100 |
21 | Rhizophagus clarus Walker & Schuessler | 8.39 | 8.33 | 100 |
22 | Rhizophagus intraradices Walker & Schuessler | – | 3.43 | 50 |
23 | Unidentified sp.2 | – | 2.45 | 50 |
Un-mined site derived inoculum showed the highest Shannon-Wiener species diversity index in both the trap cultures (2.73 in Z. mays and 2.94 in O. sativa trap culture) whereas 2 years abandoned site derived inoculum showed the least (2.17 in Z. mays and 2.25 in O. sativa) (Fig. 3). In case of Simpson’s dominance index, 10 years abandoned site derived inoculum has the highest value in Z. mays trap culture and 2 years abandoned site derived inoculum has the highest value in O. sativa trap culture (0.12 and 0.13 respectively) whereas the un-mined site derived inoculum has the least (0.07 in Z. mays and 0.06 in O. sativa trap culture) (Fig. 4). In both Z. mays and O. sativa trap cultures, un-mined site derived inoculum has the highest Pielou’s Evenness Index value (0.96 and 0.95 respectively) (Fig. 5).
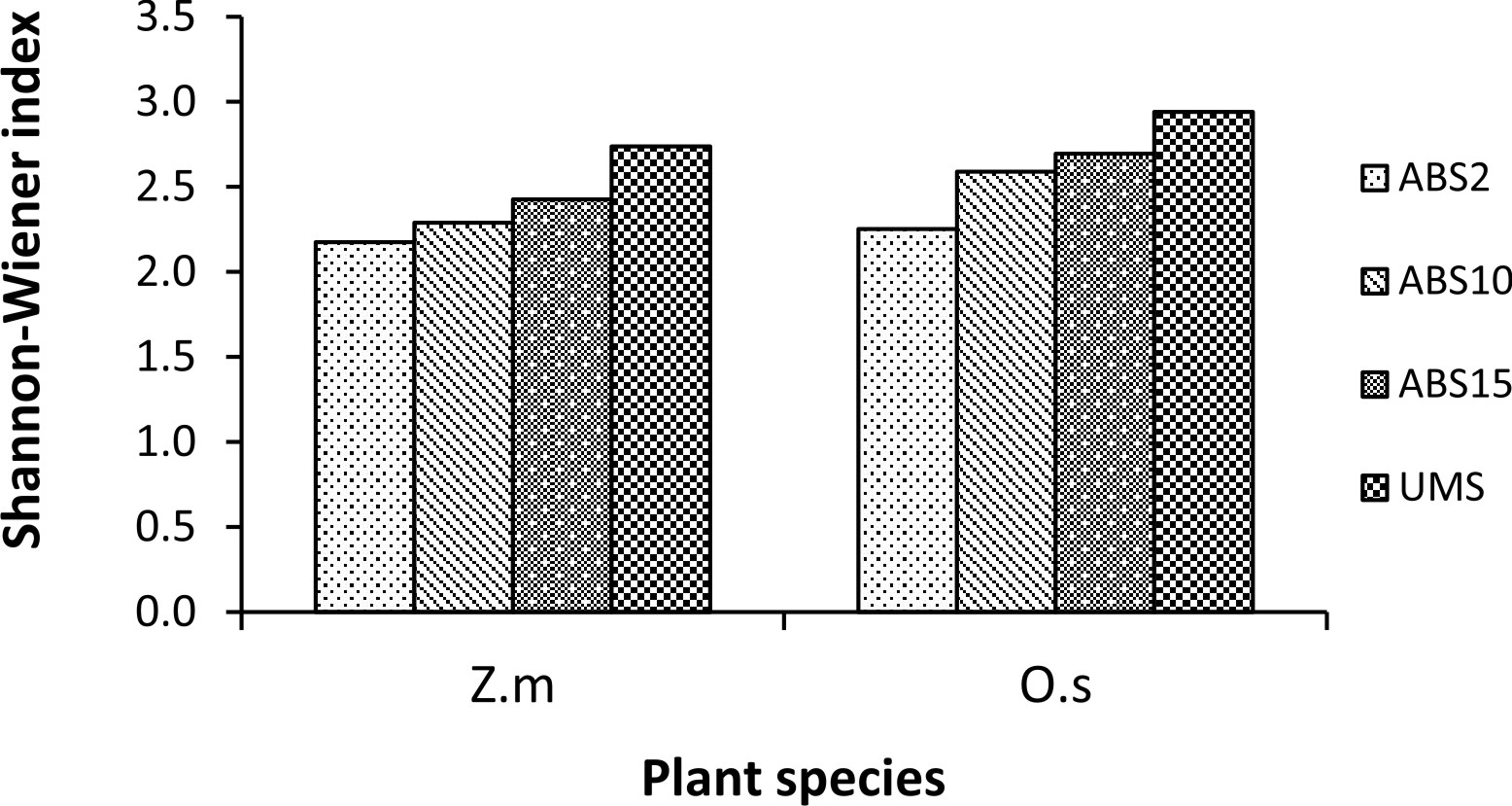
Fig. 3. Shannon-Wiener diversity index in trap culture set up with study sites derived inocula where Zea mays L. (Z.m) and Oryza sativa L. (O.s) are used as trap plants.
In Zea mays trap culture AMF colonization was least with 2 years abandoned site derived inoculum and highest with un-mined site derived inoculum whereas, in Oryza sativa trap culture AMF colonization was lowest with 2 years abandoned site derived inoculum and highest with 15 years abandoned site derived inoculum. However, spore density was least with 2 years abandoned site derived inoculum and highest with un-mined site derived inoculum in both the trap cultures. This indicates that AMF colonization level is not always directly related to spore density and is often poorly related to sporulation capacity in the soil21. Further, spore production cannot be explained by Mycorrhizal colonization level22.
Spore density showed an increasing trend with the increase in age of overburden spoils as reported earlier23-25. The number of AMF species isolated was found to be higher than those reported by Husin et al.26 and increases with age of overburden spoils which is due to vegetation development and soil physical and chemical properties27, 28.
In our present investigation, five genera of AMF were isolated (Acaulospora, Claroide-oglomus, Funneliformis, Glomus and Rhizophagus) where, Acaulospora and Glomus were dominant which is in consistent with the findings of Choudhury et al.29 and Singh and Jamaluddin 24, where they found these two genera to be dominant on spoil of all age groups. Glomus and Acaulospora species have short sporulation time and high competitive interaction and adaptability as compared to that of Gigaspora and Scutellospora species in the same environment, allowing them to establish better than the others30, 31. Acaulospora species are often associated with acidic soil32 and a wide range of host species33. 22 AMF species belonging to five genera and one unidentified species were isolated from the trap culture with un-mined site derived inoculum which is comparable to the findings of Songachan and Kayang34 from pine forest of Meghalaya.
The AMF species with 100% isolation frequency indicate that they are more tolerant to soil disturbances. Shannon-Wiener Diversity index was found to be highest in trap culture with un-mined site derived inoculum and lowest in trap culture with 2 years abandoned site derived inoculum. The diversity index is influenced by AMF species composition and relative abundance35. 2 years abandoned site derived inoculum showed the highest Simpson’s dominance index (D), indicating dominance by a few species of AMF while the lower dominance index in un-mined site derived inoculum indicates shared dominance of many AMF species.
The specific trap plants may also play an important role in sporulation and abundance of AMF spores. In our study, comparing the two trap plants, Oryza sativa showed higher colonization percentage, spore density and diversity index indicating that it has a capability to develop maximum interaction with study site derived inoculum. The host plant species has an influence on the fungal development in trap cultures36, 37. Spore density of AMF communities in trap culture depends on the host plants used38 and their number grown in each pot. The availability of roots for colonization influences sporulation by different fungal species39. AMF host preference has been observed by various authors in different plant species40, 41. Such host preferences have obvious implications for establishing efficient and comprehensive AMF trap cultures, especially for studying AMF communities in ecosystems9.
The number of AMF species isolated from the trap cultures increases with age of the coal mine overburden spoils. This indicates that with time the abandoned overburden spoils harbour a large number of AMF species. The trap cultures produced healthy spores which can be used to establish monospecific cultures for inoculum conservation. Funneliformis geosporus, Acaulospora morrowae and Acaulospora mellea were the species with highest relative abundance and can be utilized for further studies in soil reclamation.
The authors are thankful to UGC, New Delhi for financial assistance and to the Head, Centre for Advanced Studies in Botany, North-Eastern Hill University for providing laboratory facilities to carry out this work.
The authors declare that they have no conflict of interest.
- Frank AB. Ueber die auf Wurzelsymbiose beruhende Ernהhrung gewisser Bהume durch unterirdische Pilze. Berchte der Deutschen Botanischen Gesellschaft, 1885; 3:128–145.
- Feddermann N, Roger FR, Boller T, Elfstrand M. Functional diversity in arbuscular mycorrhiza—the role of gene expression phosphorous nutrition and symbiotic efficiency. Fungal Ecology, 2010; 3: 1–8.
- Smith SE, Read DJ. Mycorrhizal symbiosis. 3rd ed. Academic Press: Harcourt Brace & Company, Publishers. San Diego, London, New York, Boston, Sydney, Tokyo, Toronto, 2008.
- Miransari M. Arbuscular mycorrhizal fungi and nitrogen uptake. Archives of Microbiology, 2011; 193: 77-81.
- Siddiqui ZA, Pichtel J. 2008. Mycorrhizae: an overview. In: Siddiqui, Z.A., Akhtar, M.S., Futai, K. (eds.) Mycorrhizae: Sustainable Agriculture and Forestry. Springer, New Delhi. pp. 1-36.
- Raviv M. The use of mycorrhiza in organically-grown crops under semi arid conditions: a review of benefits, constraints and future challenges. Symbiosis, 2010; 52: 65-74.
- Miransari M. Interactions between arbuscular mycorrhizal fungi and soil bacteria. Applied Microbiology and Biotechnology, 2011; 89: 917–930.
- Van der Heijden MGA, Klironomos JN, Ursic M, et al. Mycorrhizal fungal diversity determines plant biodiversity, ecosystem variability and productivity. Nature, 1998; 396: 69–72.
- Oehl F, Sieverding E, Ineichen K, et al. Impact of land use intensity on the species diversity of arbuscular mycorrhizal fungi in agroecosystem of Central Europe. Applied and Environmental Microbiology, 2003; 69(5): 2816–2824.
- Mehrotra DM. Mycorrhizae of India forest trees. ICFRE, New Forest, Dehradun, 1991. pp. 179-184.
- Quoreshi AM. The use of mycorrhizal biotechnology in restoration of disturbed ecosystem. In: Siddiqui, Z.A., Akhtar, M.S, Futai, K. (Eds.). Mycorrhizae: Sustainable agriculture and forestry. Springer, Oxford, 2008; pp 303-320.
- Gai JP, Christie P, Feng G, Li XL. Twenty years of research on community composition and species distribution of arbuscular mycorrhizal fungi in China: a review. Mycorrhiza, 2006; 16: 229–239.
- Bever JD, Morton JB, Antonovics J, Schultz PA. Host dependent sporulation and species diversity of arbuscular mycorrhizal fungi in a mown grassland. Journal of Ecology, 1996; 84: 71–82.
- Brundrett MC. Mycorrhizas in natural ecosystems. Advances in Ecological Research, 1991; 21: 171-173.
- Muthukumar T, Udaiyan, K. Seasonality of vesicular arbuscular mycorrhizae in sedges in a semi-arid tropical grassland. Acta Oecologica, 2002; 23: 337–347.
- Sturmer SL. Effect of different fungal isolates from the same mycorrhizal community on plant growth and phosphorus uptake in soybean and red clover. Revista Brasileira de Ciencia do Solo, 2004; 28: 611–622.
- Morton JB, Bentivenga SP, Bever JD. Discovery, measurement, and interpretation of diversity in arbuscular endomycorrhizal fungi (Glomales, Zygomycetes). Canadian Journal of Botany, 1995; 73: 25-32.
- Phillips JM, Hayman DS. Improved procedures for clearing roots and staining parasitic and vesicular–arbuscular mycorrhizal fungi. Transactions of the British Mycological Society, 1970; 55: 158–160.
- McGonigle TP, Miller MH, Evans DG, et al. A new method which gives an objective measure of colonization of roots by vesicular-arbuscular mycorrhizal fungi. New Phytologist, 1990; 115: 495–501.
- Gerdemann JW, Nicolson TH. Spores of mycorrhizal Endogone species extracted from soil by wet sieving and decanting. Transactions of the British Mycological Society, 1963; 46: 235–244.
- Boddington CL, Dodd JC. The effect of agricultural practices on the development of indigenous arbuscular mycorrhizal fungi. II. Studies in experimental microcosms. Plant and Soil, 2000; 218: 145–157.
- Brundrett MC, Abbott LK, Jasper DA. Glomalean mycorrhizal fungi from tropical Australia I. Comparison of the effectiveness and specificity of different isolation procedures. Mycorrhiza, 1999; 8: 305–314.
- Jamaluddin, Chandra KK. 2009. Mycorrhizal establishment and plant succession in coal mine overburden. In: Chaubey, O.P., Bahadur, V. and Shukla, P.K. (Eds). Sustainable Rehabilitation of Degraded Ecosystems. Aavishkar publishers, distributors, Jaipur. pp.157-163.
- Singh AK, Jamaluddin. Status and diversity of arbuscular mycorrhizal fungi and its role in natural regeneration on limestone mined spoils. Biodiversitas, 2011; 12 (2): 107-111.
- Carrenho R, Trufem SFB, Bononi VLR. Fungos micorrizicos arbusculares em rizosferas de tres especies de fitobiontes instaladas em area de mata ciliar revegetada. Acta Botanica Brasilica, 2001; 15: 115-124.
- Husin EF, Khairul U, Zakir Z, Emalinda O. Spores Diversity of Arbuscular Mycorrhizal Fungi and Their Use for Land Reclamation in Coal Mining Used Land. Der Pharmacia Lettre, 2017; 9 (2): 79-86.
- Herrmann L, Lesueur D, Brau L, et al. Diversity of root-associated arbuscular mycorrhizal fungal communities in a rubber tree plantation chrono-sequence in Northeast Thailand. Mycorrhiza, 2016; 26: 863–877.
- Kruger C, Kohout P, Janouskova M et al. Plant Communities Rather than Soil Properties Structure Arbuscular Mycorrhizal Fungal Communities along Primary Succession on a Mine Spoil. Frontiers in Microbiology, 2017; 8: 1-16.
- Choudhury B, Kalita MC, Azad P. Distribution of arbuscular mycorrhizal fungi in marshy and shoreline vegetation of Deepar Beel Ramsar Site of Assam, India. World Journal of Microbiology and Biotechnology, 2010; 26: 1965–1971
- Nandakwang P, Elliott S, Dell B, et al. Arbuscular mycorrhizal status of indigenous tree species used to restore seasonally dry tropical forest in Northern Thailand. Research Journal of Microbiology, 2008; 3: 51–61.
- Singh S, Pandey A, Chaurasia B, Palni LMS. Diversity of arbuscular mycorrhizal fungi associated with the rhizosphere of tea growing in ‘natural’ and ‘cultivated’ ecosites. Biology and Fertility of Soils, 2008; 44: 491–500
- Teixeira-Rios T, De Souza RG., Maia LC, et al. Arbuscular mycorrhizal fungi in a semi-arid, limestone mining-impacted area of Brazil Acta Botanica Brasilica, 2013; 27(4): 688-693.
- Straker CJ, Hilditch AJ, Rey MEC. Arbuscular mycorrhizal fungi associated with cassava (Manihot esculenta Crantz). South African Journal of Botany, 2010; 76: 102–111.
- Songachan LS, Kayang H. Diversity of arbuscular mycorrhizal fungi in pine forest of Meghalaya, North East India. Mycosphere, 2011; 2(4): 497–505.
- Yang AN, Lu L, Zhang N. The diversity of arbuscular mycorrhizal fungi in the subtropical forest of Huangshan (Yellow Mountain), East-Central China. World Journal of Microbiology and Biotechnology, 2011; 27: 2351-2358.
- Brundrett MC, Jasper DA, Ashwath N. Glomalean mycorrhizal fungi from tropical Australia II. The effect of nutrient levels and host species on the isolation of fungi. Mycorrhiza, 1999; 8: 315–321.
- Jansa J, Mozafar A, Anken T, et al. Diversity and structure of AMF communities as affected by tillage in a temperate soil. Mycorrhiza, 2002; 12: 225–234.
- Lovelock CE, Andersen K, Morton JB. Arbuscular mycorrhizal communities in tropical forests are affected by host tree species and environment. Oecologia, 2003; 135: 268–279.
- Ahulu EM, Gollote A, Gianinazzi-Pearson V, Nonaka M. Co-occurring plants forming distinct arbuscular mycorrhizal morphologies harbor similar AM fungal species. Mycorrhiza, 2006; 17: 37–49.
- Skorovב Z, Wiemken A, Redecker D. Co-occurring Gentiana verna and Gentiana acaulis and their neighboring plants in two Swiss upper montane meadows harbor distinct arbuscular mycorrhizal fungal communities. Applied and Environmental Microbiology, 2007; 73: 5426–5434.
- Leal PL, Sturmer SL, Siqueira JO. Occurrence and diversity of arbuscular mycorrhizal fungi in trap cultures from soils under different land use systems in the Amazon, Brazil. Brazilian Journal of Microbiology, 2009; 40: 111-121.
© The Author(s) 2019. Open Access. This article is distributed under the terms of the Creative Commons Attribution 4.0 International License which permits unrestricted use, sharing, distribution, and reproduction in any medium, provided you give appropriate credit to the original author(s) and the source, provide a link to the Creative Commons license, and indicate if changes were made.